Dr Clare Sansom, Senior Associate Lecturer in Birkbeck’s Department of Biological Sciences reports on a recent, international capacity-building workshop to tackle antimicrobial resistance and accelerate new antibiotic discovery, sponsored by the global challenges research fund (GCRF).
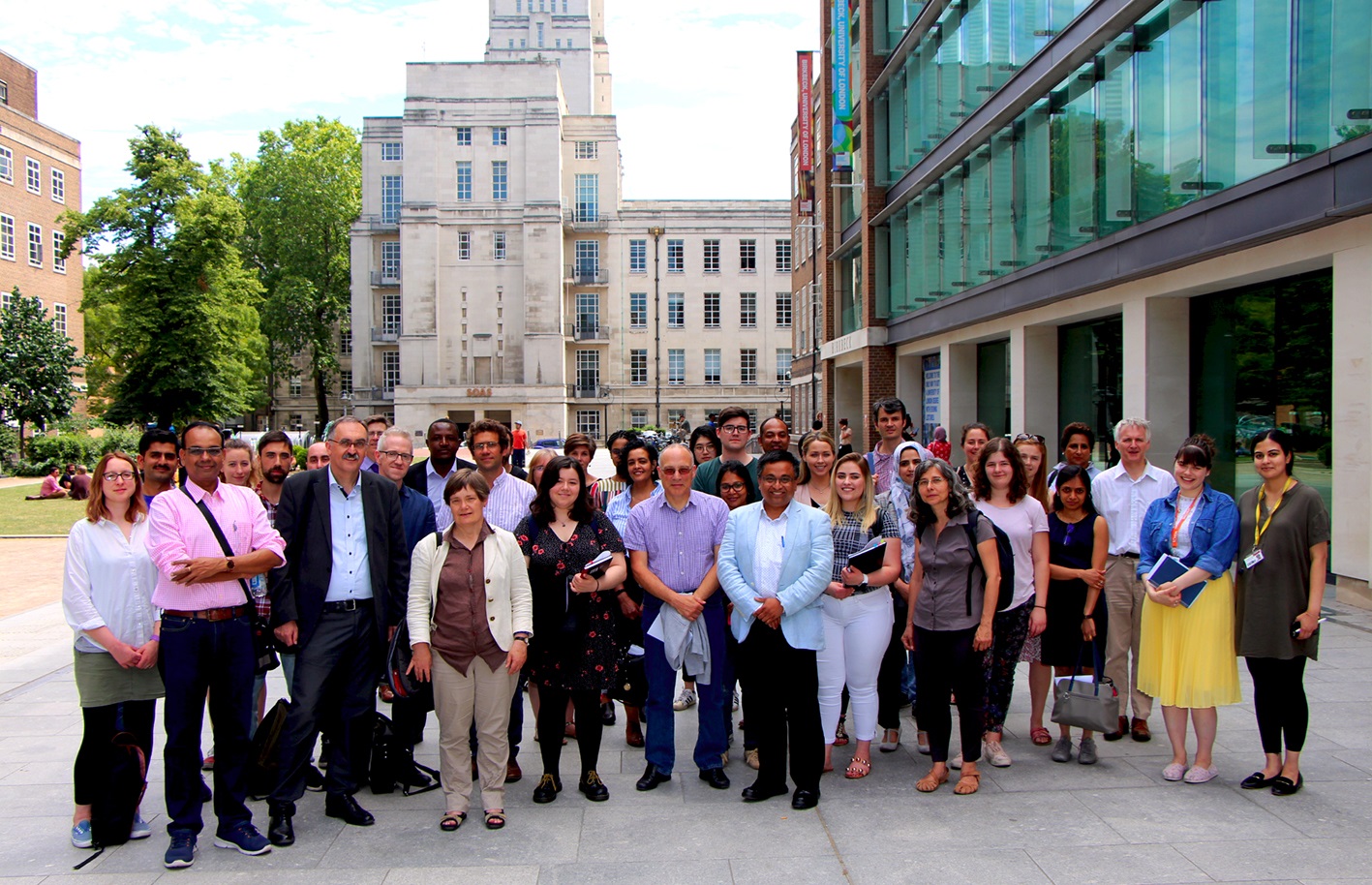
Credit: Mr Harish Patel
Antibiotic resistance is one of the most serious threats to global health: some commentators have even rated it as important as climate change. It is, however, one that the research community – particularly in the academic and not-for-profit sectors – is finally investing serious resources in tackling. Research into novel antibiotic targets and the compounds that can interact with them is burgeoning throughout the world. At Birkbeck, research in the ISMB-Microbiology Research Unit, headed by Professor Sanjib Bhakta focuses on tackling antibiotic resistance in priority bacterial pathogens, the causative agent of a number of global infectious diseases.
In July 2019 Prof Bhakta invited his collaborators in the UK and overseas to a workshop at Birkbeck to discuss ways of tackling drug resistance. This was funded through the UK’s Grand Challenges Research Fund (GCRF), an initiative to promote the welfare of developing countries through international research. Delegates were welcomed by Birkbeck’s Pro-Vice-Master for internationalism, Professor Kevin Ibeh, and then heard brief presentations from Dr Sarah Lee on the place of the GCRF in Birkbeck’s research portfolio and Dr Ana Antunes-Martins on the mission of the neighbouring London International Development Centre. This combines the resources of seven University of London institutions in the Bloomsbury area, including Birkbeck, to support interdisciplinary research, capacity building and public engagement for international development.
In an intense scientific session, the first researcher to speak was Professor Nicholas Keep, Executive Dean of the School of Science at Birkbeck and a structural biologist. He presented some structures of Mycobacterium tuberculosis proteins known or believed to be novel prospective therapeutic targets that had been solved at Birkbeck or UCL using the three main techniques of structural biology: X-ray crystallography, nuclear magnetic resonance (NMR) and electron microscopy (EM). These included a small enzyme called resuscitation-promoting factor, which is necessary for the bacteria to emerge from their dormant state, and a rather larger one that synthesises an important component of the cell wall. This work is enabled by excellent local facilities including a new Titan Krios microscope in Professor Helen Saibil’s EM lab, and even more powerful national and international ones.
Beta-lactamase enzymes, which inactivate drugs in the penicillin family, are one of the most common antibiotic resistance mechanisms, but they are not as well understood in mycobacteria as they are in some other human pathogens. Prof Anindya S. Ghosh from the Indian Institute of technology in Kharagpur – incidentally, the speaker who had travelled the furthest – described his group’s work in collaboration with Prof Bhakta and Prof Tabor’s lab (funded by a Newton-Bhabha international fellowship to Sarmistha Biswal at Birkbeck this year) in designing inhibitors for beta-lactamases and other proteins that interact with penicillins and prevent their antibiotic action. He set out several more opportunities for collaborative research, including finding molecules that prevent the formation of drug-resistant microbial biofilms.
The next two speakers came from continental Europe, and both described novel natural sources of potential anti-infective drugs. Prof Franz Bucar from the University of Graz, Austria focused on drugs from plant and fungal sources, including an intriguingly named flavonoid, skullcapflavone II (derived from the poisonous skullcap mushroom). This and other recently discovered natural products were also highlighted on a poster by Prof Bucar’s doctoral student, Julie Solnier. Prof Ester Boix from Universitat Autònoma de Barcelona in Spain described how the antimicrobial peptides that we synthesise as a defence against bacteria might be harnessed as drugs. Her group has used the HT-SPOTi assay developed in Prof Bhakta’s group at Birkbeck to screen human ribonuclease peptides against macrophages infected with Mycobacterium tuberculosis.
Dr Jody Phelan and Prof Taane Clark from the London School of Hygiene and Tropical Medicine asked – and answered – the question ‘What can the M. tuberculosis genome tell us about drug resistance in TB?” This bacterial genome contains over 4 million base pairs of DNA and about 4,000 genes, compared to the 3.3 billion base pairs and 20,000 genes of the human genome. Resistance to any of the over 10 drugs currently used to treat the disease arises largely when treatment is irregular or stopped too soon, and the genetic changes responsible for each type of resistance can be identified rapidly using whole genome sequencing. It is now possible to use this in clinical practice to predict which drugs a given strain is most likely to be resistant to, and thence to recommend a personalised course of treatment for an individual patient.
Dr Simon Waddell from the University of Sussex in Brighton described how the RNA molecules transcribed from the M. tuberculosis genome change during the lifecycle and with the environment of the bacterium, and how this analysis, known as transcriptomics or RNA profiling, can both track and predict responses to drug therapy. One new compound, a benzothiazinone discovered through a high-throughput screen, was found to induce transcription from the same set of genes as cell-wall synthesis inhibitors, suggesting that it is likely to act against the bacterium through the same or a similar mechanism.
These two ‘omics talks were followed by two extremely short ones by scientists based in the Department of Chemistry at University College London. Dr Rachael Dickman (from Prof Alethea Tabor’s lab in UCL) is developing potential antibacterial agents based on a complex amino acid, lanthionine. These ‘lantibiotics’ bind to Lipid II, which is formed during cell wall synthesis, and therefore act as inhibitors of that synthesis. Professor Helen Hailes described the antimicrobial properties of a series of isoquinolines that selectively inhibit slow-growing mycobacteria and that may also potentiate the activity of other drugs by preventing their efflux from bacterial cells.
With the last talk, by Prof Matthew Todd of the UCL School of Pharmacy, the workshop moved from pure science to begin to discuss the economics of drug discovery. Prof Todd’s open source drug discovery work, which began with a project on malaria, is completely open: all the data is freely available, all ideas are shared, no results are ‘owned’ by any of the researchers and there will be no patents. It is a timely approach and one that can involve anyone – Prof Todd has recently been awarded a grant by the Royal Society to work with teenagers at Sevenoaks School to develop new antifungals – and one that might, perhaps, help any of the academic groups represented at the workshop turn their novel ideas into drugs that are useful against the killer disease.
The final networking session was accompanied by an engaging talk by me, Dr Clare Sansom, about the important issue of communicating the challenge of antimicrobial resistance to non-scientists. This was illustrated with frightening scenes from fictional accounts of possible post-antibiotic futures and included a quiz that many of the experts present found surprisingly challenging. This workshop was organised in collaboration with the Commonwealth Scholarships Commission, UK and approved by the Royal Society of Biology for continual professional development credit.